Chapter 11, “Dual Nature of Radiation and Matter,” explores the wave-particle duality of radiation and matter, which is a fundamental concept in quantum mechanics. The chapter begins by discussing the photoelectric effect, where light behaves as a particle (photon) to eject electrons from a metal surface. This phenomenon could not be explained by classical wave theory, leading to the introduction of the concept of photons by Albert Einstein. The chapter then delves into the wave nature of particles, as proposed by de Broglie, who suggested that particles such as electrons also exhibit wave-like behavior. This idea was confirmed through experiments like electron diffraction, which demonstrated that electrons can produce interference patterns, a characteristic of waves.
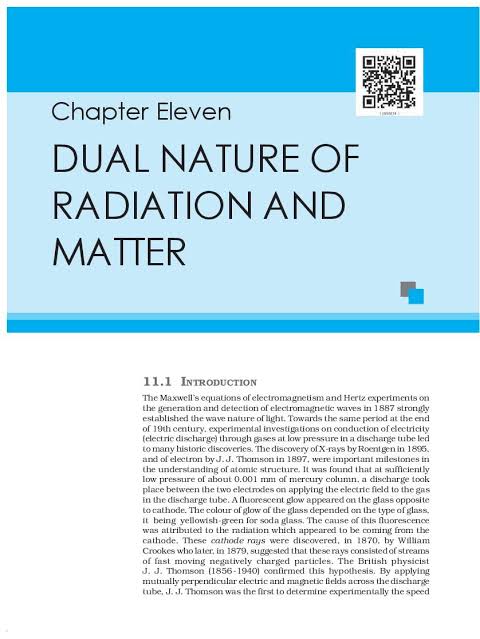
Class 12th Physics Chapter 11 Dual Nature of Radiation and Matter Book 📚 download in PDF format 👇👇
The chapter also covers Heisenberg’s Uncertainty Principle, which states that it is impossible to precisely measure both the position and momentum of a particle simultaneously. This principle highlights the limitations of classical physics in describing the behavior of subatomic particles and underscores the probabilistic nature of quantum mechanics. Additionally, the chapter touches on the applications of wave-particle duality in technologies like electron microscopes, which utilize the wave nature of electrons to achieve higher resolution imaging.
Overall, Chapter 11 provides a comprehensive understanding of how light and matter exhibit both wave and particle characteristics, challenging the traditional notions of physics and laying the groundwork for modern quantum mechanics. This dual nature has profound implications for the behavior of particles at the atomic and subatomic levels and is essential for understanding the principles of quantum mechanics.
Short and Long Answers
1. What is the photoelectric effect?
Short Answer: The photoelectric effect is the emission of electrons from a metal surface when it is exposed to light of sufficient frequency. This effect demonstrates the particle nature of light.
Long Answer: The photoelectric effect refers to the phenomenon where electrons are emitted from a metal surface when it is exposed to light of a frequency higher than a certain threshold value. According to classical wave theory, the energy of light depends on its intensity, but the photoelectric effect could only be explained by assuming that light consists of particles called photons, each carrying a quantum of energy. Albert Einstein proposed that the energy of these photons is proportional to the frequency of light (E = hν). When a photon strikes the metal surface, its energy is transferred to an electron, and if this energy exceeds the work function of the metal, the electron is ejected. The photoelectric effect provided crucial evidence for the particle nature of light and led to the development of quantum theory.
2. State de Broglie’s hypothesis.
Short Answer: De Broglie’s hypothesis states that particles like electrons have a wave nature, and the wavelength associated with a particle is inversely proportional to its momentum.
Long Answer: De Broglie hypothesized that just as light exhibits both wave and particle characteristics, matter (such as electrons) also has a dual nature. He proposed that every particle with momentum (p) has an associated wavelength (λ), given by the relation λ = h/p, where h is Planck’s constant. This idea was revolutionary because it extended the concept of wave-particle duality to all matter, not just radiation. De Broglie’s hypothesis was later confirmed by experiments like electron diffraction, where electrons were observed to create interference patterns, a property typically associated with waves. This wave nature of particles is a cornerstone of quantum mechanics and has significant implications in understanding the behavior of subatomic particles.
3. What is Heisenberg’s Uncertainty Principle?
Short Answer: Heisenberg’s Uncertainty Principle states that it is impossible to simultaneously determine the exact position and momentum of a particle with absolute precision.
Long Answer: Heisenberg’s Uncertainty Principle is a fundamental concept in quantum mechanics that states there is an inherent limit to the precision with which certain pairs of physical properties, such as position and momentum, can be known simultaneously. Mathematically, it is expressed as Δx·Δp ≥ ħ/2, where Δx is the uncertainty in position, Δp is the uncertainty in momentum, and ħ is the reduced Planck’s constant. This principle arises from the wave-like nature of particles and implies that the more accurately we measure one of these quantities, the less accurately we can know the other. The Uncertainty Principle challenges the deterministic nature of classical physics and introduces the concept of probability in the behavior of particles.
4. Explain the concept of matter waves.
Short Answer: Matter waves refer to the wave-like behavior of particles, as described by de Broglie’s hypothesis, which states that particles have an associated wavelength.
Long Answer: The concept of matter waves, introduced by Louis de Broglie, suggests that particles such as electrons exhibit wave-like properties in addition to their particle characteristics. According to de Broglie, a particle with momentum p has an associated wavelength λ = h/p, where h is Planck’s constant. This wave nature of particles was experimentally verified by observing the diffraction and interference patterns produced by electrons and other particles, phenomena that are typically associated with waves. The concept of matter waves is fundamental to quantum mechanics, as it explains the dual nature of matter and helps describe the behavior of particles at the quantum level, including the formation of atomic orbitals and the nature of chemical bonds.
5. What is the significance of the Compton effect?
Short Answer: The Compton effect is the scattering of X-rays by electrons, leading to a change in wavelength, and it provides evidence for the particle nature of electromagnetic radiation.
Long Answer: The Compton effect, discovered by Arthur Compton, is the phenomenon where X-rays scatter off electrons, resulting in a change in the wavelength of the X-rays. This change in wavelength depends on the angle of scattering and is explained by treating X-rays as particles (photons) that collide with electrons. The Compton effect provided significant evidence for the particle nature of electromagnetic radiation, supporting Einstein’s theory of photons. The effect also demonstrated the conservation of energy and momentum in photon-electron interactions, further validating the quantum theory of light. The Compton effect is important in the study of quantum mechanics and has applications in medical imaging and the study of atomic and molecular structures.
6. What is the work function of a metal?
Short Answer: The work function is the minimum energy required to eject an electron from the surface of a metal.
Long Answer: The work function of a metal is the minimum amount of energy needed to remove an electron from the surface of the metal to a point just outside the metal, where the electron is free from the influence of the metal’s positive ions. This energy barrier varies for different metals and is a crucial factor in the photoelectric effect. When light of a certain frequency or higher is incident on a metal surface, the photons transfer their energy to the electrons. If this energy is greater than the work function of the metal, electrons are emitted from the surface. The concept of the work function is essential in understanding the photoelectric effect and is used in applications such as photoelectric cells and electron microscopy.
7. Describe the experimental verification of de Broglie’s hypothesis.
Short Answer: De Broglie’s hypothesis was experimentally verified by observing electron diffraction, where electrons produced interference patterns, indicating their wave nature.
Long Answer: De Broglie’s hypothesis, which suggested that particles such as electrons have an associated wavelength, was experimentally verified through electron diffraction experiments. In these experiments, electrons were directed at a crystal, and the resulting pattern was observed on a screen. The electrons produced an interference pattern, similar to that produced by waves passing through a double slit. This observation confirmed that electrons, and by extension other particles, exhibit wave-like behavior, validating de Broglie’s hypothesis. The wave nature of electrons has since become a fundamental concept in quantum mechanics, influencing the development of technologies such as electron microscopes and the understanding of atomic structure.
8. What is the significance of Planck’s constant?
Short Answer: Planck’s constant is a fundamental constant that relates the energy of a photon to its frequency and is central to the theory of quantum mechanics.
Long Answer: Planck’s constant (h) is a fundamental constant in quantum mechanics that relates the energy of a photon to its frequency (E = hν). It has a value of approximately 6.626 x 10^-34 Js. Planck’s constant plays a crucial role in the quantization of energy levels in atoms and the dual nature of light and matter. It was first introduced by Max Planck in his explanation of blackbody radiation, where he proposed that energy is quantized and can only be emitted or absorbed in discrete amounts called quanta. This concept laid the foundation for the development of quantum theory and has profound implications for our understanding of the microscopic world, including the behavior of electrons in atoms and the properties of light.
9. What is the principle behind an electron microscope?
Short Answer: An electron microscope uses the wave nature of electrons to achieve high-resolution imaging of small objects.Long Answer: The principle behind an electron microscope is based on the wave nature of electrons, as described by de Broglie’s hypothesis. Electrons, having a much shorter wavelength than visible light, can achieve much higher resolution when used to image small objects. In an electron microscope, a beam of electrons is focused onto a sample, and the interaction of the electrons with the sample produces an image with a much higher resolution than what is possible with optical microscopes. The shorter wavelength of electrons allows the electron microscope to resolve features at the atomic level, making it an essential tool in materials science, biology, and nanotechnology.
Get involved!
Comments